The engineering team at Avangard Company, relying on over two decades of experience and advanced academic education from reputable universities both inside and outside the country, as well as the production of more than ten thousand parts for industries such as machinery manufacturing, automotive, mold making, oil and gas, petrochemicals, power plants, cement, and mineral processing, with alloys including carbon steels, low-alloy steels, heat-resistant steels (stainless and refractory), manganese steels (Hadfield), high-chromium steels, and chromium cast irons, is also capable of producing parts based on specific analyses, standards, and desired characteristics requested by our esteemed customers. Using advanced engineering software and a well-equipped metallography laboratory, along with other mechanical tests, and by defining appropriate solidification gradients and other necessary processes, the requested part is delivered to you with a valid certificate, the best quality, and the most competitive price.
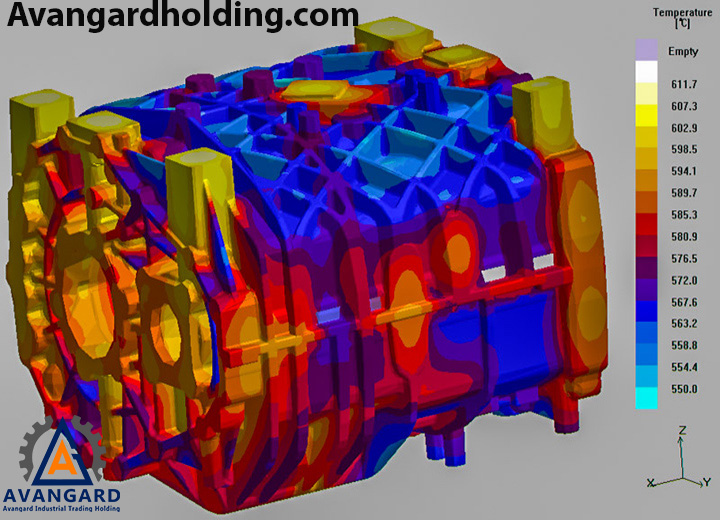
In fact, since humans gained the ability to melt and extract metals, the phenomenon of solidification has been a part of human knowledge and skill in manufacturing tools and equipment. Solidification is considered one of the most economical methods for producing many metals.
In recent decades, with the development of human knowledge in understanding materials and the impact of production process variables on microstructure and mechanical properties, the importance of solidification in metal casting has increased significantly. The solidification rate of the melt determines the final microstructure of the part, and the microstructure of the part determines its properties. For this reason, in some cases, heaters and coolers are installed around the mold to regulate the solidification rate and ensure uniform solidification in the part. It is important to note that the cooling rate varies in different areas of the part.
Related topic: “Casting Products”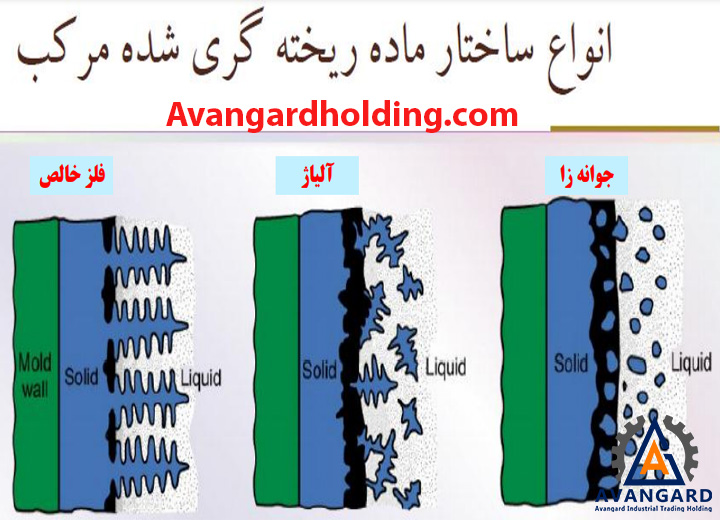
Currently, for analyzing solidification phenomena in casting, on one hand, various metallurgical approaches and techniques are being developed to control and improve solidification microstructures. On the other hand, using powerful computers for numerical analysis and engineering simulations, behaviors such as heat transfer, fluid flow, and phase transformations during solidification are being studied and evaluated for various parts and alloys.
Solidification varies depending on which of the following types of metal melting is involved:
- A pure element
- An alloy
- Solidification of pure metals
Due to the high heat transfer at the mold wall, a thin shell of solidified metal forms immediately at the interface between the mold wall and the molten metal. In this shell, due to the high cooling rate, the microstructure consists of fine and randomly oriented grains. As solidification progresses, the grains elongate in the direction of heat transfer.
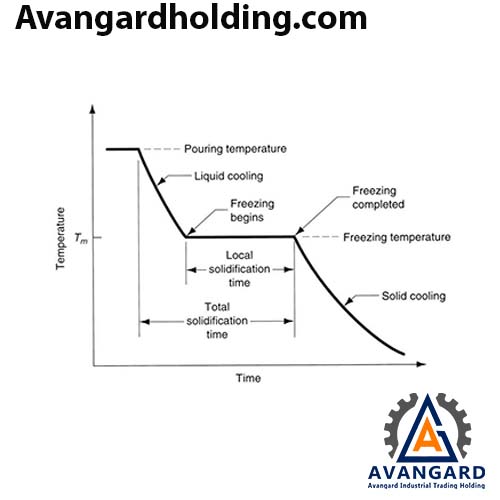
Most alloys solidify over a range of temperatures rather than at a single point. Solidification begins at the molten temperature and continues until the solidification temperature is reached. Like pure metals, a thin shell of solidified metal forms immediately at the interface between the molten metal and the mold wall.
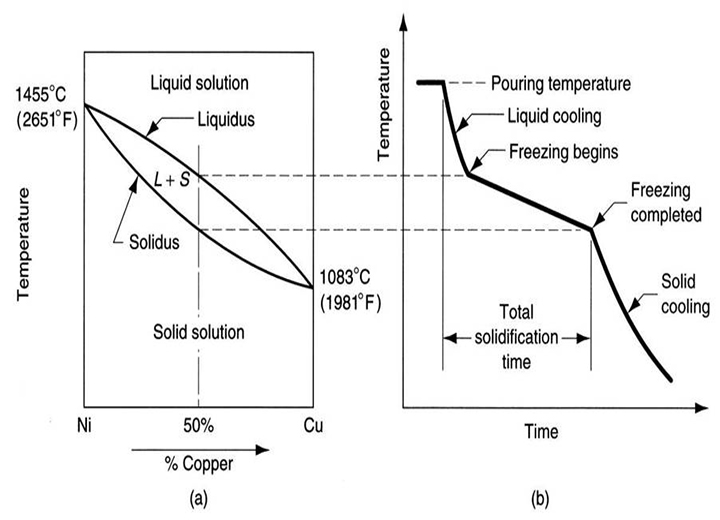
Dendrites gradually form. However, due to the wide temperature range between the solidus and liquidus lines, part of the molten metal solidifies earlier, and its grains grow more in the direction of heat transfer compared to the part that cools later. As a result, the molten metal at the center of the part cools more slowly, leading to a finer-grained structure.
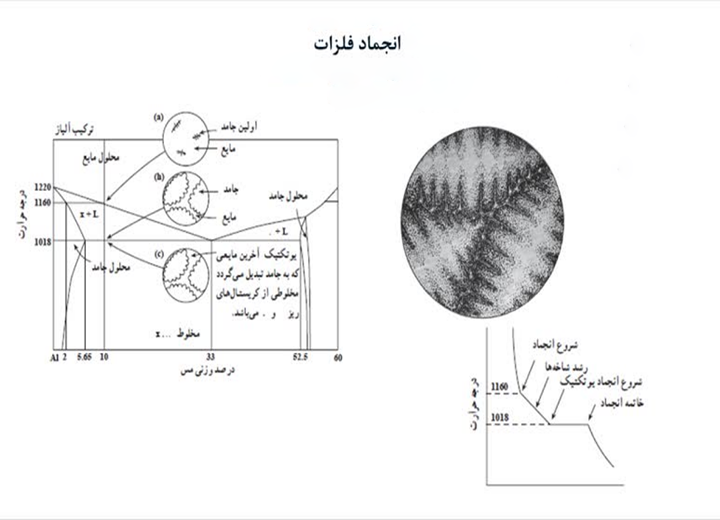
- During the cooling of the metal in the molten state
- During cooling from the liquid to the solid state
- During cooling of the solid metal to ambient temperature
The effect of the solidification rate of molten metal in casting is important from various perspectives, such as its impact on production, metal structure, mechanical properties, and more. Increasing the solidification rate causes the molten metal in the mold to solidify in a shorter time, allowing more parts to be produced within a specific timeframe. Therefore, if the solidification rate of the molten metal in the mold can be increased in a way that does not adversely affect the properties of the part, production quantities can be increased, and more parts can be produced at a lower cost, making it economically advantageous. On the other hand, the solidification rate can alter the properties and microstructure of the part. For instance, if the solidification rate, or in other words, the cooling rate of the molten metal, is increased, a large number of solid nuclei (solid seeds) form in the melt, which, upon further growth, result in a fine-grained microstructure. This structure exhibits relatively good mechanical properties. In such a structure, due to the fine grains, the number of grain boundaries increases, requiring greater force and stress to deform the fine-grained structure. As a result, the part demonstrates higher strength. Additionally, the fine-grained structure enhances toughness and flexibility, allowing the metal to exhibit good resistance to mechanical forces.
Related topic: “Design and modeling in casting”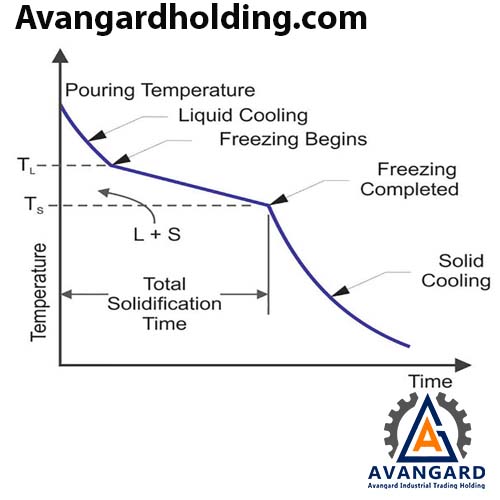
If the solidification cooling rate, or the cooling rate of the molten metal, decreases, the number of solid nuclei (seeds) will be minimized. In this case, the growth of the nuclei continues, resulting in a coarse-grained structure. In parts with a coarse-grained microstructure, due to the lower number of grain boundaries, the strength is less compared to parts with a fine-grained structure. However, at high temperatures, a part with a coarse-grained structure exhibits better resistance to applied forces compared to a part with a fine-grained structure.
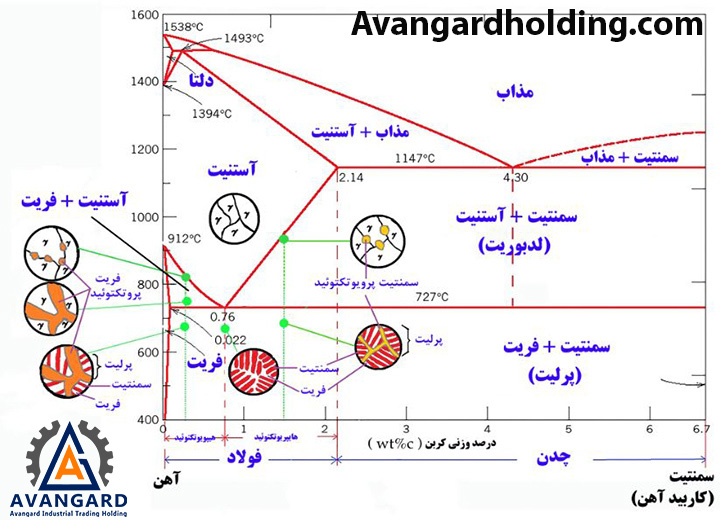
The solidification rate of the melt depends on the thermal capacity and thermal conductivity of the molten metal and the material of the mold. The higher the thermal conductivity and thermal capacity of the molten metal and the mold material, the faster the heat is transferred outward, and the molten metal solidifies more quickly. For example, molten metal in metal or graphite molds cools and solidifies faster than in sand molds.
The best type of solidification in casting is directional or controlled solidification, where the solidification of the molten metal starts from the farthest parts of the mold relative to the feeder and the final stage of solidification occurs in the feeder. Another method for directional solidification is the use of chills, which are typically made of materials such as copper, steel, graphite, and aluminum. Chills are usually placed inside the mold cavity or on its walls, referred to as internal chills or external chills, respectively. Sometimes, in certain parts of the mold, the use of sands such as chromite sand is recommended during molding due to their high cooling capacity. Chills create a higher temperature gradient, directing the solidification front and thereby increasing the feeding range, especially in alloys with pasty solidification. Additionally, in all alloys, the direction of solidification can be controlled using chills or layering (insulating part of the mold). In this case, shrinkage cavities and porosity form in the feeder. On the other hand, gases and impurities in the molten metal are pushed toward the feeder by the solidification front and are expelled from the part. After solidification, by removing the feeder, a high-quality part is obtained.
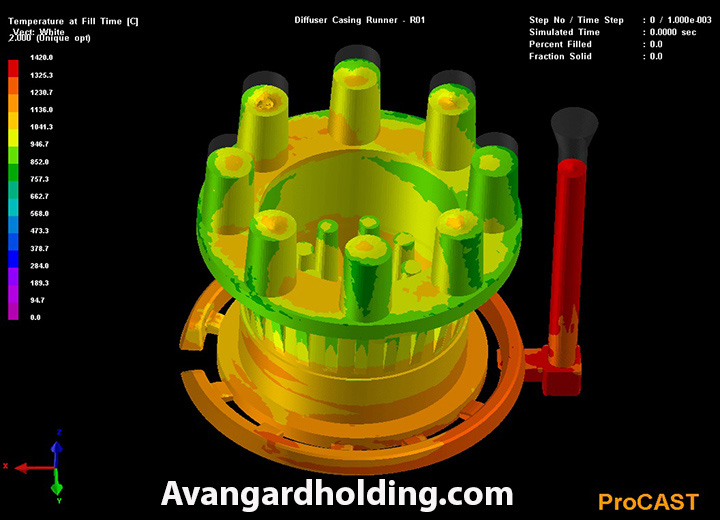
Chill Zone:
This zone has very fine grains due to the contact of the molten metal with the mold surface.
Columnar Growth Zone:
In this zone, grains form in a columnar structure, and the orientation of the grains is always aligned with their crystalline direction and opposite to the direction of heat transfer. Columns that are more aligned with the heat transfer direction move competitively and block the growth of adjacent cells. If the pouring temperature increases, the likelihood of longer columns increases due to the higher thermal gradient. If the pouring temperature decreases, the length of the columns decreases due to the reduced gradient.
Equiaxed Zone:
Due to the decrease in temperature and increase in concentration, the combined undercooling in the center of the part increases, allowing homogeneous nucleation and growth to occur. Additionally, the competitive growth of columns can lead to the detachment of parts of the columns, which are then ejected into the molten front.
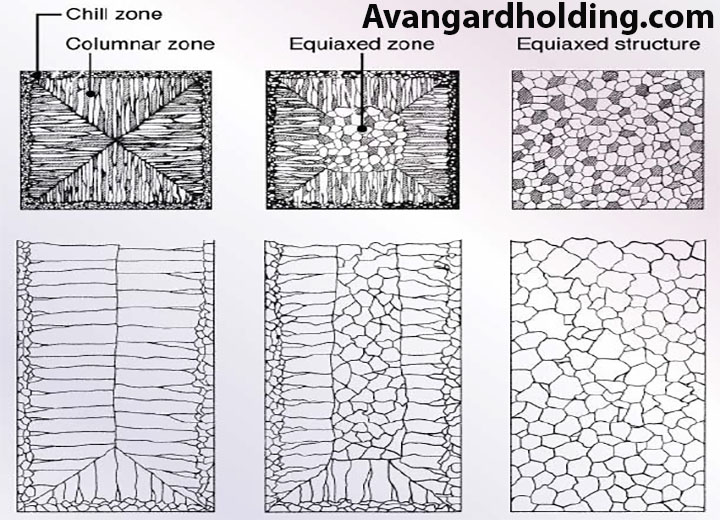